The intriguing question of whether one could escape a blender by jumping out of it has sparked curiosity and debate among many. The answer lies in understanding the principles of physics and the relationship between mass, height, and jumping ability. Interestingly, the key insight into this conundrum can be traced back to the 17th-century observations of Alfonso Borelli, a pioneer in biomechanics. Borelli noticed an intriguing pattern: animals of diverse sizes seemed to possess similar jumping capabilities, regardless of their mass or height. This observation set the stage for a fascinating inquiry into human performance within the constraints of the blender’s walls, which are approximately 15 times our height! Despite the significant differences in mass and stature among dogs, cats, horses, and squirrels, they all astonishingly jump around the same vertical distance of roughly 1.2 meters. This phenomenon can be explained by the law that states the energy generated by our muscles is directly proportional to our mass. In other words, the harder we push or pull, the more force we generate, regardless of our size. While it may seem counterintuitive, this principle holds true for all animals, including humans. Despite being much larger and heavier, we can jump similar heights as smaller creatures because our muscles produce energy on a scale proportional to our mass. This explanation provides a logical answer to the puzzle, suggesting that simply jumping is the key to escaping the blender. However, there’s a twist! If one were shrunk down to the size of a nickel, their strength-to-weight ratio would become vastly superior. This heightened ratio would enable them to generate tremendous force relative to their small mass, allowing for impressive vertical leaps. Nonetheless, size matters when it comes to leg length and the time your feet spend pushing against the ground during a jump. If one’s legs are extremely short, as they would be at a reduced scale, the contact time with the ground is disproportionately short, leading to an inefficient jump. This dynamic highlights the challenge of escaping the blender even with enhanced strength. Instead, a more strategic approach might involve using one’s increased strength to bend and twist the blades of the blender like a flexible spring, potentially creating a path of escape. In conclusion, while the idea of jumping out of a blender may seem whimsical, it invites us to explore the fascinating interplay between physics, biology, and human capabilities. It serves as a reminder that understanding underlying principles can provide insight and inspire innovation, even in unexpected contexts.
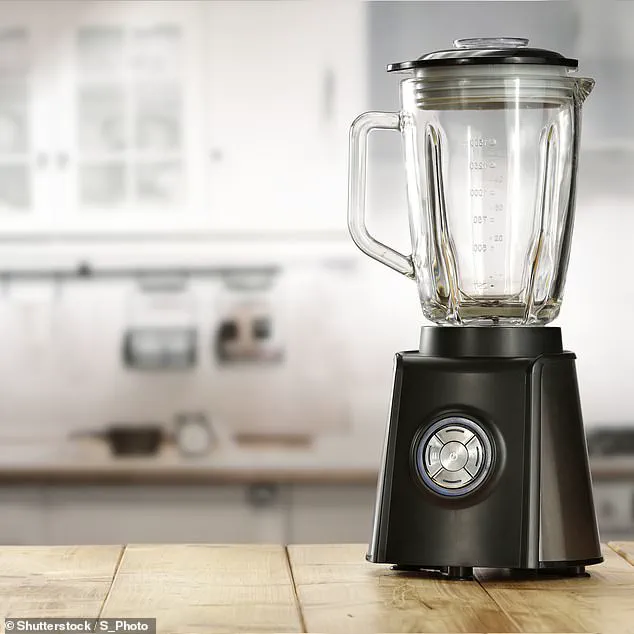
Storing energy and then releasing it is a clever way to achieve a powerful jump, as explained by Professor Gregory Sutton, an expert on insect motion from the University of Lincoln. He told MailOnline that muscle produces mechanical energy, which can accelerate animals up to certain heights. ‘If that animal is half the size, it has half the energy but also half the mass, so it actually jumps to the same height,’ he noted. This concept can be seen in action with grasshoppers, where two grasshoppers holding hands can jump a metre high, while a million grasshoppers doing the same would reach the same height due to the increased mass and muscle force.
Professor Sutton further explained that animal muscles work by contracting fibres called sarcomeres all at once, creating movement. The more sarcomeres pulling at the same time, the greater the force generated. This idea has been noted since the 17th century, and it holds true for animals with similar body plans. For example, dogs, horses, and squirrels can all jump around a metre in the air because jump height doesn’t scale to body size.
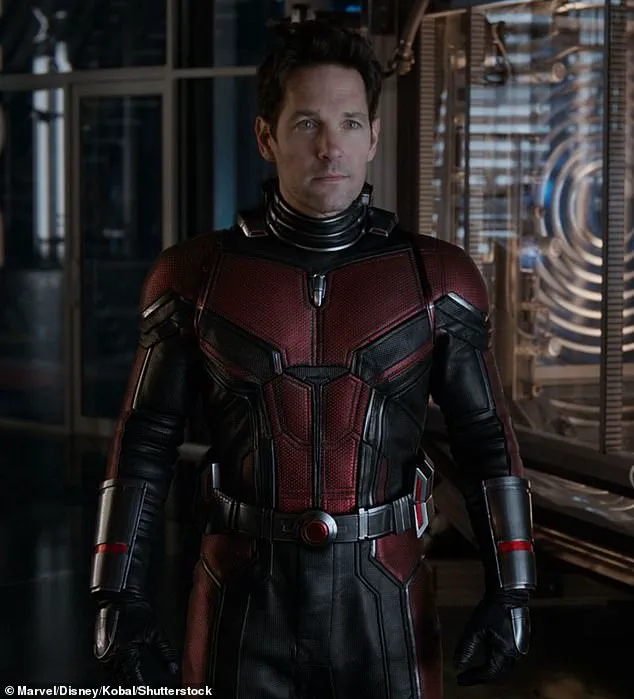
The key takeaway is that by carefully controlling muscle activity, animals can achieve impressive jumps despite their size. This understanding of animal movement has implications for robotics and human performance, offering potential improvements in both areas.
For those who don’t already know, jumping high is all about transferring as much energy from your legs into the ground before you leave it. However, this gets progressively harder as we get smaller and shorter! This got us thinking: why does size matter when it comes to jumping height? And so, we set out to find an answer.
We picture two people of different heights jumping on a trampoline – let’s call them Tall Tim and Short Sam. When it’s time for their big bounce, both start from a crouch, but the differences in their bodies quickly become apparent. For Tim, his long legs give him that extra second or two to gather speed before he takes off; this is crucial in helping him reach higher heights. On the other hand, Sam is faced with a different challenge. Even when starting from a low position, he has to work faster to achieve the same height as Tim. The problem lies in the shorter time he has to build up energy and transfer it into the ground.
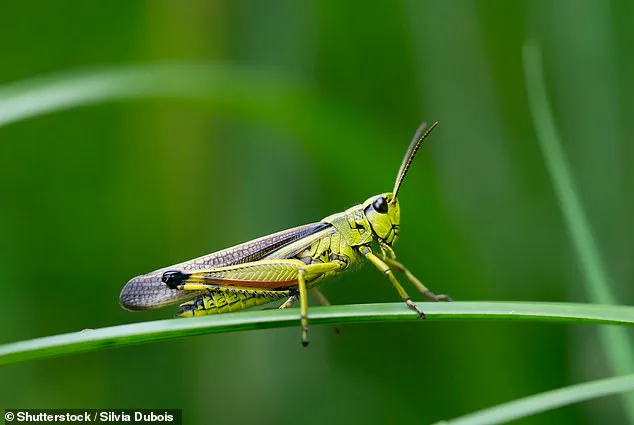
But why does this matter? Well, it all boils down to muscle contraction speed. Sam’s muscles need to work even harder than Tim’s to create the same amount of upward force over a much shorter period of time. This is where being smaller really makes a difference – those tiny muscles have their work cut out for them! So, if you ever find yourself short on height but big on ambition, don’t worry; it’s all about working with your body’s natural advantages and maximizing every second of your jump. Who knows, maybe one day we’ll see a new world record set by someone who’s mastered the art of super-speed muscle contraction!
It’s an intriguing thought experiment that brings to light the importance of body proportions in athletic performance. While we may not be able to change our height, understanding these underlying mechanics can help us optimize our jumps and achieve new heights (no pun intended). So, the next time you see someone jumping high, remember that it’s not just about height but also about how well your body can harness energy during that crucial fraction of a second.
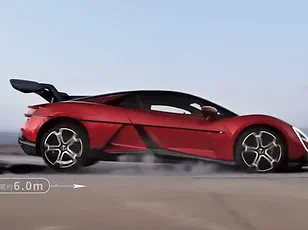
In conclusion, we hope this story has provided some insight into the fascinating world of human physics and jump dynamics. Stay tuned for more intriguing articles on the science behind sports!
A fascinating insight into the world of miniature humans and their unique jump capabilities has been revealed by biomechanics expert Dr. Maarten Bobbert from the Vrije Universiteit Amsterdam. In a recent interview with MailOnline, Dr. Bobbert shed light on the unexpected limitations faced by these tiny beings when attempting to jump.
A new study has revealed that while tiny animals may not be able to jump as high as their larger counterparts, they make up for it with longer jumping distances. The galago bush baby, for example, can jump 12 times its body length, but dedicates a whopping 30-40% of its body mass to leg muscles, giving it the power to leap great distances. This is in contrast to a human coin-size body, which could only manage a pitiful 5-10 cm in the air, even with a rubber band ‘catapult system’ as suggested by Professor Sutton. The key difference in strength-to-mass ratio between tiny and larger creatures highlights the advantages of being small when it comes to jumping heights, but not distances. This study adds to the fascinating world of animal adaptations and offers unique perspectives on problem-solving, even in miniature forms.
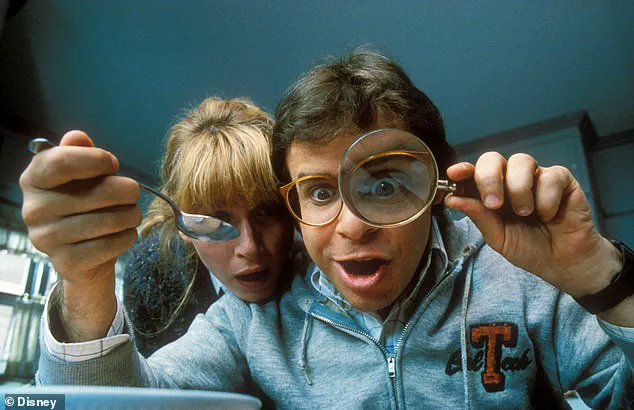
Insects are known for their incredible jumping abilities, defying the limits of their small bodies. But how do they manage to leap such great distances? The secret lies in a clever biomechanical trick. As Professor Jim Usherwood, an expert on motion mechanics, explains, insects like grasshoppers use their leg muscles not just to move but also to charge up springs built into their legs. This innovative approach bypasses the traditional force-velocity trade-off that muscles face. By winding up these tiny springs, the insects can store a significant amount of energy and then release it in a fraction of a second, propelling themselves forward with incredible speed and accuracy. Professor Usherwood’s fascinating insight offers a new perspective on insect motion, revealing how they conquer the challenge of short limbs and weak muscles. It’s almost as if these creatures are using a spring-loaded mechanism to supercharge their jumps, making them appear faster and higher than one would expect given their physical size. This unique adaptation showcases the ingenuity of nature, where even the smallest creatures find clever ways to overcome obstacles. Professor Usherwood’s explanation is further backed up by the expertise of Professor Sutton, who provides additional context to this intriguing phenomenon. Together, these scholars shed light on the fascinating world of insect movement, revealing how they have mastered the art of jumping with precision and speed. The concept of using muscle power to store energy in springs isn’t limited to insects; it can be applied to various other fields as well. For instance, engineers might draw inspiration from this natural design to create more efficient energy-storing systems for human-made machines or vehicles. As we continue to explore the wonders of nature, we may uncover even more驚異的な適應模式,這些模式可以激發人類技術的進步。
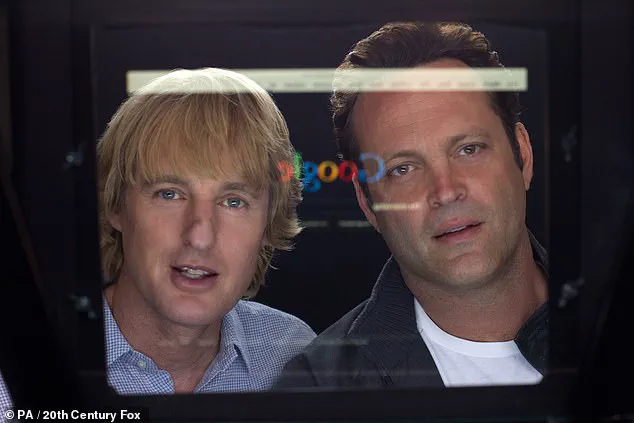
A fascinating discovery has been made in the world of insects: their ability to escape certain death with incredible agility and power. The trap jaw ant, a tiny creature with a mighty bite, has caught the attention of scientists for its unique method of self-propulsion. By utilizing spring-like tendons in its jaws, this little ant can generate an astonishing 200,000 watts of energy per kilogram, far surpassing the power output of human muscle, which hovers around 100 watts per kilogram. This discovery highlights the innovative ways nature finds to overcome physical limitations.
The trap jaw ant’s jaws act like a powerful spring-loaded mechanism. As the ant bites into the ground, the tendons store energy, and when released, they shoot the ant upward with incredible force. This technique allows the ant to leap into the air, providing it with a much-needed escape mechanism from predators or threats.
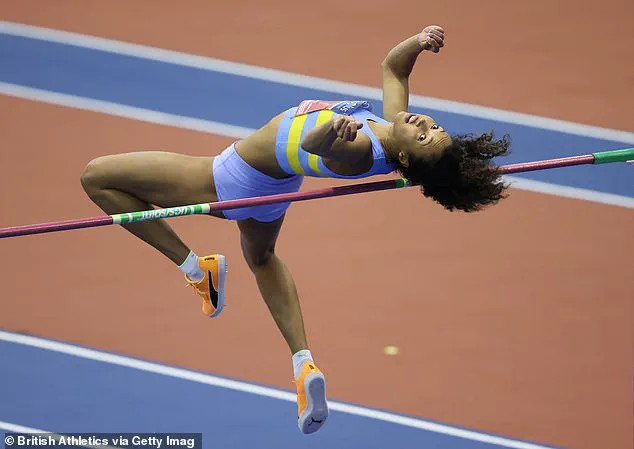
Comparing this ant’s ability to power through the Blender Puzzle by Google, where participants must find a way out of a rapidly spinning blender full of hazards, we can find inspiration for our own escape strategies. The key takeaway is not to rely solely on muscle power but to explore alternative sources of energy and propulsion methods. By bending the blades of the blender like springs or using elastic bands to launch oneself forward, one could potentially emulate the ant’s escape technique and find a way out of the puzzle.
This discovery in insect behavior not only fascinates but also inspires us to think creatively about problem-solving and movement. The trap jaw ant serves as a reminder that sometimes the most effective solutions lie in nature’s clever designs, offering us a fresh perspective on how we approach challenges and innovate for our own escape.
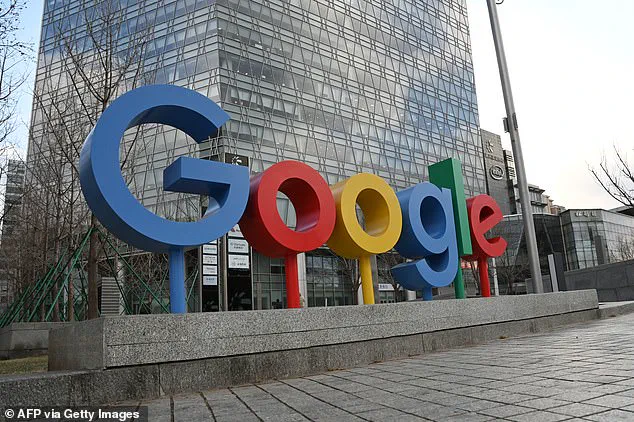
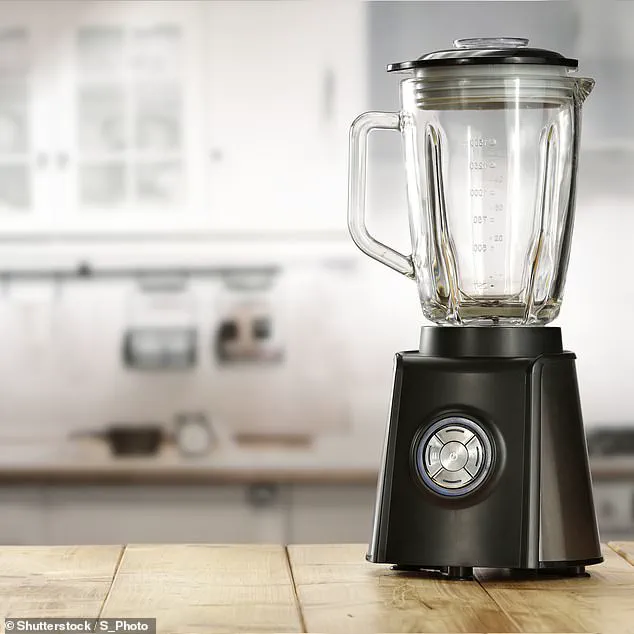
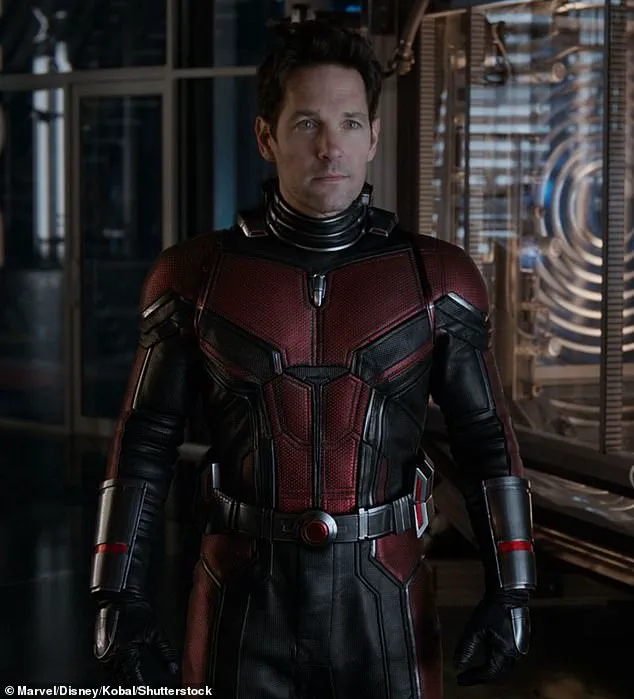
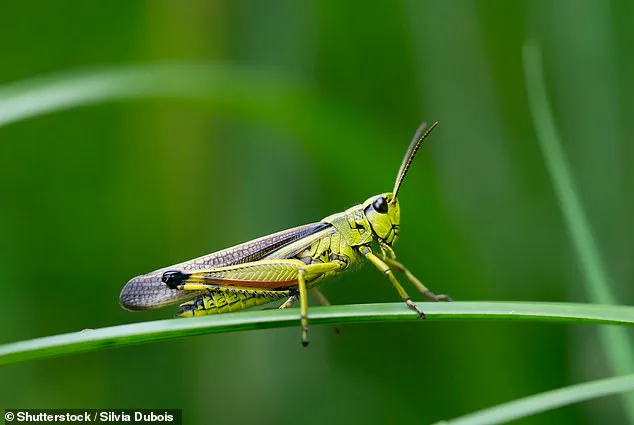
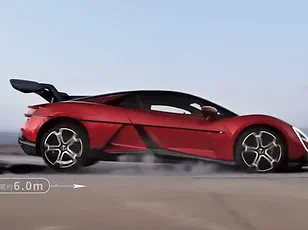
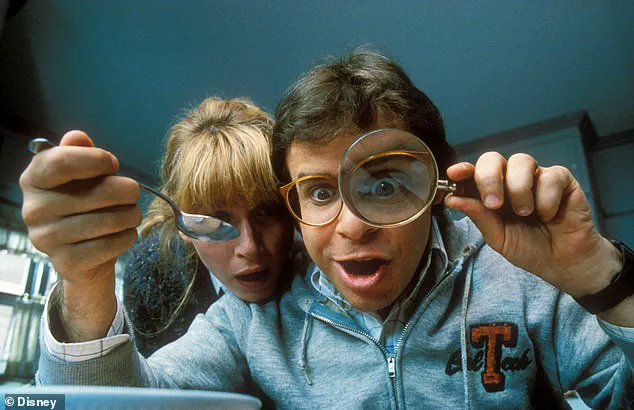
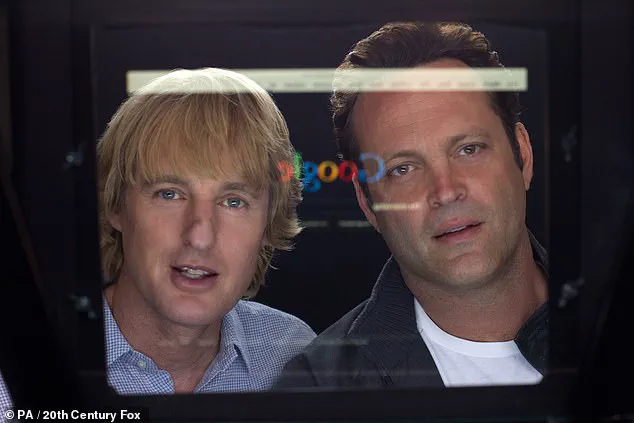
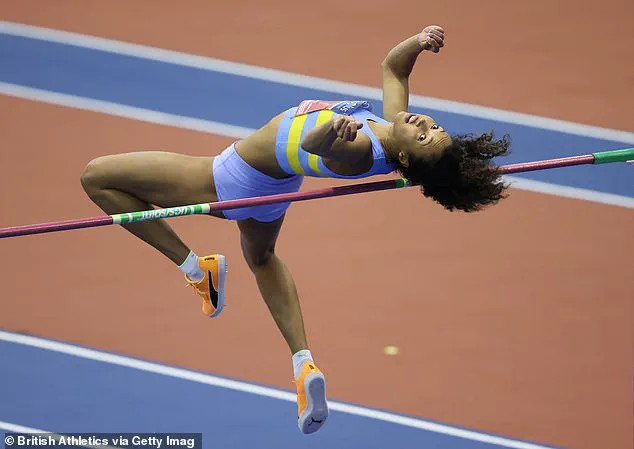
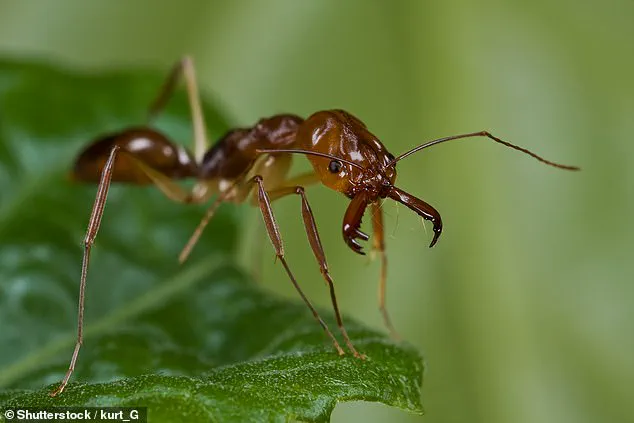
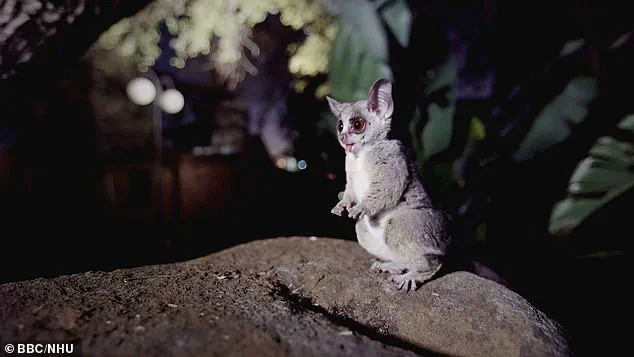
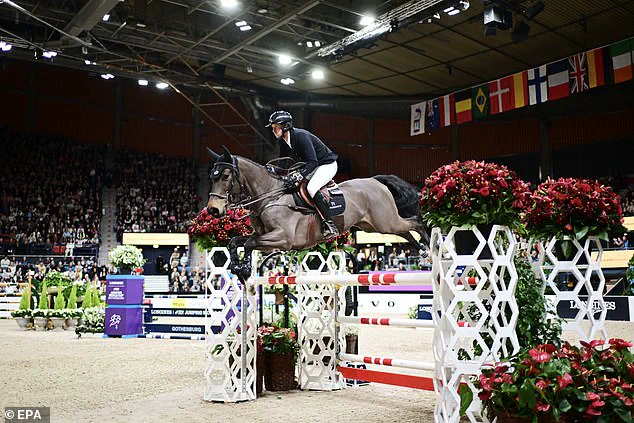